Endurance Events and Lactate Testing
With Emphasis on the Triathlon
15 of 19
Anaerobic metabolism is the gatekeeper for aerobic energy
Model of Energy Metabolism
The following is a description of the model of energy metabolism developed by Alois Mader. It is not in any textbook despite there being thousands of books that discuss energy metabolism. Yet it describes a scenario that fits the data better than any of the theories in the textbooks. The lactate threshold or maximal lactate steady state is acknowledged but its origins are said to be a mystery. It is no mystery. It is the result of a few basic forces playing off against each other producing results that are easy to see and described by Mader's model.
The basic idea that is missing from current energy metabolic theories is how the aerobic and anaerobic systems interact. And despite the very large majority of the energy being delivered from aerobic energy, something else controls this delivery. This control or, as we express it, a gatekeeping process is the anaerobic system. An athlete may have a very large aerobic capacity as indicated by a VO2 max test but he or she may not be able to use it to the extent they wish. The following is a little more technical than other things the reader will see on this topic but it explains this gatekeeping effect.
Mader model - Pyruvate/lactate formation at a given effort level depends on three variables. These three variables are:
- VO2 max - this is aerobic capacity or the maximum rate of energy production by the aerobic system. VO2 max is usually estimated by a device attached to the mouth and nose of an athlete. The device measures oxygen uptake by athletes as they complete a progressive exercise test to exhaustion.
- VO2 steady state - this is the amount of aerobic energy that is being used during a sub-maximal steady state exercise. It is usually measured using the same instruments as for VO2 max.
- VLamax - this is anaerobic capacity or the maximum rate of energy production by the glycolytic system. It is sometimes designated by the term Plamax or maximum production of lactate. In reality this is the maximum rate of production of pyruvate and lactate but since lactate is what is measured “La” has been used for this term.
The theory states that VLa (the production of lactate) at any steady state level is a function of VO2 max, VO2 at that level and VLamax.
VLa = f(VO2 max, VO2 steady state, VLamax)
For example, suppose a runner has the following aerobic and anaerobic capacities (VO2 max of 60 ml/kg/min and maximum lactate production rate of .7 mmol/sec/l.)
This chart below shows a lactate/pyruvate production curve based on these capacities. This is not the typical lactate curve you have seen throughout this set of modules on endurance sports and the triathlon, even though it is deceptively similar in shape. The curves you normally see for lactate tests are measures of lactate levels in the blood, usually plotted against a measure of speed or power. This is an estimate of lactate production not lactate levels and it is in the muscle fiber itself. Notice the measures are different. The left axis is lactate production, not lactate levels. The unit of lactate production is lactate mmols per minute per liter. It is a rate such as miles per hour or meters per second. The total lactate production capability for this athlete is 42 mmol per minute per liter based on the estimated maximum production level of .7 mmol/sec/l. So this chart indicates that the athlete will never get close to the maximum production level.
The lower axis is VO2 for the current steady state effort level.
Remember our formula from just above, VLa = f(VO2 max, VO2 steady state, VLamax). The VLa is the left axis in the chart. The VO2 steady state is the bottom axis. The VO2 max and VLamax are constants for each athlete and remain the same for this testing protocol. As the intensity of the exercise rises and is measured by the VO2 measured at each stage, the rate of lactate production will also increase. The red curve measures the rate of lactate production.
Thus, as the athlete increases in speed and increases his oxygen uptake, the amount of lactate produced also starts to rise. At first it will rise very slowly but as the runner increases speed not only will his oxygen uptake increase but so will the production of lactate. So at very low levels the anaerobic system that produces the lactate is being used only sparingly but at higher effort levels, the percentage of energy supplied by anaerobic energy increases and then reaches a point where it starts to increase very rapidly. Notice the anaerobic capacity of this athlete is 42 mmol per min per liter (VLamax of .7 mmol/sec/l x 60 seconds) but on this chart he never gets close to that capacity as production rates range from less than 1% of anaerobic capacity to about 7.1% of anaerobic capacity as he approaches 80% of aerobic capacity (48 ml/kg/min).
The next chart compares two athletes with different aerobic capacities but equal anaerobic capacities. It is similar to the preceding chart except the new athlete has a VO2 max of 75 ml/kg/l. The lactate/pyruvate production curve is lower at every steady state VO2. Increasing the VO2 max and keeping the maximum lactate production rate the same, produces less lactate.
So the amount of lactate produced by the glycolytic system depends not only on the strength of the anaerobic system (VLamax) but also on the strength of the aerobic system. As we pointed out earlier the muscle must choose how to replenish the ATP and as the aerobic capacity increases it chooses to replace more of the ATP by aerobic processes at every effort level.
At a steady state of 40 ml/kg/l the athletes with the greater aerobic capacity (75 ml/kg/l) will produce less than half the lactate of the athlete with the lower aerobic capacity (60 ml/kg/l)
With athletes that have similar aerobic capacities but different anaerobic capacities the muscle again will make different choices on how it replaces the ATP. In the chart below three athletes with different anaerobic capacities but the same aerobic capacity (60 ml/kg/l) produce substantially different amounts of lactate.
The athlete with an anaerobic capacity of .1 mmol/s/l will produce very little lactate until he gets close to VO2 max and then his lactate production will shoot up dramatically. The athlete with an anaerobic capacity of 1.3 mmol/s/l will produce substantial amounts of lactate even at low effort levels. So as anaerobic capacity rises or falls the muscle chooses different systems to replace ATP.
The lower the maximum lactate production rate, the further to the right is the curve. This shows why marathoners want to have a low VLamax. The lower it is the less lactate will be produced and the higher percentage of VO2 max they can run. However, they will not have any speed for acceleration or speed at the end. Nor would they be very good at 1500 m. Remember our comparison of the three runners. A runner with a higher VLamax and the same VO2 max will be faster at 1500 m.
An aside: actually a marathoner will not want a VLamax as low as .1 mmol/s/l (extremely low) because the body will not produce enough lactate or pyruvate to fuel aerobic metabolism by burning carbohydrates and the muscles will have to use fat as a fuel. This will slow the athlete down, because fat metabolizes at about half the rate of pyruvate and causes the athlete to contract the muscles at a slower rate.
The lactate production rates between individuals with a VLamax of 0.1 mmol/s/l and one with 1.3 mmol/s/l is very dramatic. The next chart shows that the rate of lactate production is 10-14 times greater at a VLamax of 1.3 mmol/s/l vs. a VLamax of 0.1 mmol/s/l for three different steady state levels.
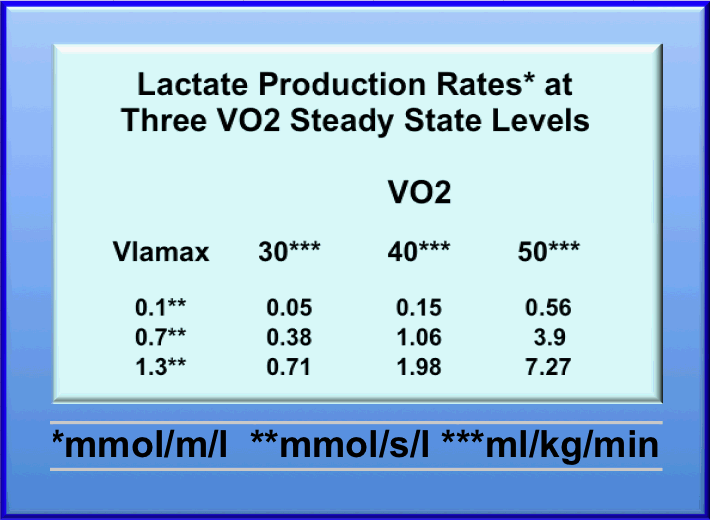
This dramatic influence of VLamax on lactate levels is not widely discussed in either the academic literature or in the popular training press. What are the implications of this for training and for performance? Continue as we discuss the anaerobic system further and discuss how to train it to help achieve an optimal performance.
Continue on to module 16 on controlling the gatekeeper
Module 16 of 19- how do you train to control the gatekeeper for aerobic energy?